Quantum Dynamics
Our research explores the quantum dynamics of many-body states in materials through theoretical modeling and numerical simulations, emphasizing connections to experimental measurements within the pump-probe framework. We concentrate our efforts on addressing the following key problems.
Pump-Probe Spectroscopy Theory and Simulations
Achieving predictive light control over quantum materials requires detailed, real-time knowledge of their nonequilibrium states on ultrafast timescales. This capability is accessed through pump-probe techniques, where a high-intensity laser pulse disrupts the equilibrium state, and a weaker probe pulse tracks the evolving response. These spectroscopies uniquely provide insights into both time and energy aspects of the material’s behavior. However, nonequilibrium conditions introduce theoretical complexities beyond equilibrium models, requiring simultaneous consideration of intrinsic correlation functions and extrinsic probe wavefunctions to accurately interpret results.
Our group is dedicated to developing rigorous microscopic theories for a range of pump-probe spectroscopies, such as time-resolved resonant inelastic x-ray scattering (trRIXS), time-resolved x-ray absorption (trXAS), time-resolved Raman scattering, and time-resolved ARPES. A central area of interest is understanding nonlinear phenomena in pump-probe experiments, including finite-lifetime effects in trRIXS, high harmonic generation, and two-photon processes. To meet the demands of studying correlated materials, we also design wavefunction-based quantum many-body methods that numerically simulate these spectroscopies, enhancing our ability to interpret and anticipate experimental findings. These methods help bridge theoretical models with cutting-edge experimental techniques, advancing our understanding of nonequilibrium quantum materials.
Relevant papers:
- Wang et al. “X-ray Scattering from Light-Driven Spin Fluctuations in a Doped Mott Insulator“, Commun. Phys. 4, 212 (2021)
- Mitrano and Wang, Probing Light-Driven Quantum Materials with Ultrafast Resonant Inelastic X-Ray Scattering. Communications Physics 3, 184 (2020)
- Wang et al. “Time-Resolved Resonant Inelastic X-Ray Scattering in a Pumped Mott Insulator“, Phys. Rev. B 101, 165126 (2020)
- Chen et al. “Theory for Time-Resolved Resonant Inelastic X-ray Scattering“. Physical Review B 99, 104306 (2019)
- Wang et al. “Theory of Time-Resolved Raman Scattering in Correlated Systems: Ultrafast Engineering of Spin Dynamics and Detection of Thermalization“, Phys. Rev. B 98, 245106 (2018)
- Wang et al. “Theoretical Understanding of Photon Spectroscopies in Correlated Materials In and Out of Equilibrium“, Nat. Rev. Mater. 3, 312 (2018)
- Wang et al. “Producing Coherent Excitations in Pumped Mott Antiferromagnetic Insulators“, Phys. Rev. B 96, 235142 (2017)
Ultrafast Characterization and Control of Entangled States
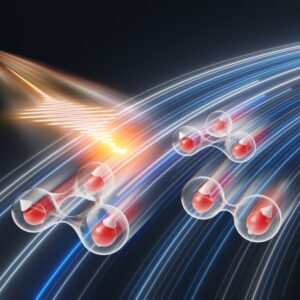
A critical question in quantum technology is how to quantify, design, and control entanglement. In quantum optics, tools like interferometry allow for direct measurement of entanglement entropy, but quantum materials lack such characterization techniques. Measuring many-body wavefunctions precisely and exerting full control over degrees of freedom remain significant challenges in this domain.
To approach these challenges, we employ entanglement witness methods, leveraging observable spectral properties to provide a lower bound on multipartite entanglement for both equilibrium and transient states. Our group’s research, grounded in pump-probe theory, focuses on linking these ultrafast spectral observables to reconstruct specific entanglement witnesses, including quantum Fisher information and cumulative reduced density matrices. By developing these connections, we aim to identify systems and mechanisms for light-controlled entanglement, opening new avenues for dynamic control.
Beyond instantaneous characterization, we investigate how nonequilibrium dynamics can reveal entangled quantum states in materials. Certain entangled states, such as quantum spin liquids, often lack clear signatures in traditional spectroscopy due to their subtle, low-energy characteristics. By harnessing the additional time-domain data captured through nonequilibrium dynamics, we aim to uncover distinctive properties of these elusive states, ultimately enhancing our ability to characterize, detect, and control complex entangled states in quantum materials.
Relevant papers:
- Jiang et al. Using Magnetic Dynamics to Measure the Spin Gap in a Candidate Kitaev Material, arXiv:2405.03212 (2024)
- Hales et al. Witnessing Light-Driven Entanglement using Time-Resolved Resonant Inelastic X-Ray Scattering, Nature Communications 14, 3512 (2023)
- Wang et al. Fluctuating Nature of Light-Enhanced d-Wave Superconductivity: A Time-Dependent Variational Non-Gaussian Exact Diagonalization Study, Physical Review X 11, 041028 (2021)
Nonthermal Control of Microscopic Interactions
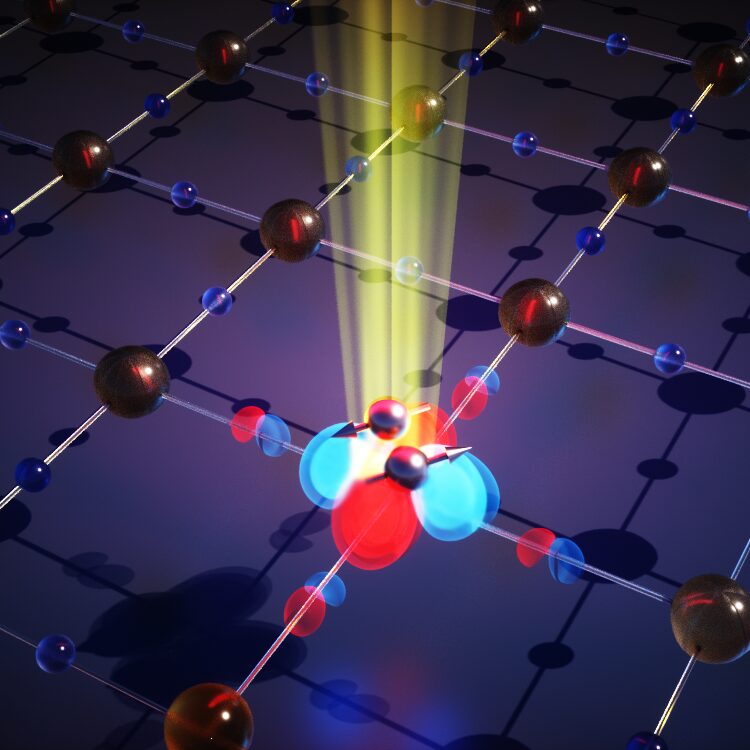
When a material is driven by a laser field, its electronic states become dressed with photons, creating a hybridized system of light and matter. Under a continuous monochromatic light field, many nonequilibrium dynamics are effectively described through Floquet theory, which simplifies complex time-dependent quantum behaviors into a superposition of steady states. In this framework, the quasi-energies of these states are offset by multiples of the photon energy, with each steady state comprising dressed electrons and photons. This laser-driven system enables precise control over single-particle dispersions and interactions.
Our group explores the mechanisms enabling light-controlled interactions in correlated materials, with a particular focus on Floquet engineering of many-body states. In such systems, light-induced interactions— including spin exchange, Coulomb interaction, electron-phonon coupling, and spin-orbit coupling —allow for dynamic control over intrinsic microscopic parameters that are otherwise rigid in equilibrium. Using unbiased, wavefunction-based simulations, we investigate optimal pump conditions and timescales for approximating and stabilizing these nonthermal states. Through pump-probe simulations, we seek to detect the distinctive spectral fingerprints that characterize these engineered states.
Relevant papers:
- Xie et al. Dynamical Approach to Realize Room-Temperature Superconductivity in LaH10, arXiv:2312.12706 (2023)
- Baykusheva et al. Ultrafast Renormalization of the Onsite Coulomb Repulsion in a Cuprate Superconductor, Physical Review X 12, 011013 (2022).
- Wang et al. “X-ray Scattering from Light-Driven Spin Fluctuations in a Doped Mott Insulator“, Commun. Phys. 4, 212 (2021)
- Chen et al. Observing Photo-Induced Chiral Edge States of Graphene Nanoribbons in Pump-Probe Spectroscopies. npj Quantum Materials 5, 84 (2020)
- Wang et al. “Theory of Time-Resolved Raman Scattering in Correlated Systems: Ultrafast Engineering of Spin Dynamics and Detection of Thermalization“, Phys. Rev. B 98, 245106 (2018)
- Wang et al. “Producing Coherent Excitations in Pumped Mott Antiferromagnetic Insulators“, Phys. Rev. B 96, 235142 (2017)
Light-Induced Superconductivity
Superconductivity represents a critical quantum phase with transformative applications in energy-efficient transport, medical imaging, and quantum computing. Yet, achieving coherent Cooper pairing typically demands extreme conditions, such as ultra-low temperatures or high pressures, which limit practical implementations. Recent advances in ultrafast laser techniques offer promising avenues to transiently modify electronic structures and many-body interactions, raising the potential to induce superconducting states at temperatures and pressures beyond established critical thresholds.
Our group investigates nonequilibrium strategies to stabilize superconductivity as metastable excited states. We are interested in both conventional superconductivity, where electron-phonon coupling is the primary pairing mechanism, and unconventional superconductivity driven by strong electronic correlations. To enable these investigations, we develop numerical methods that capture dynamics involving both strong correlations and robust electron-phonon couplings, facilitating a detailed study of potentially light-induced superconducting phases.
Relevant papers:
- Xie et al. Dynamical Approach to Realize Room-Temperature Superconductivity in LaH10, arXiv:2312.12706 (2023)
- Tang et al. Orbitally Selective Resonant Photodoping to Enhance Superconductivity, Physical Review B 104, 174516 (2021)
- Wang et al. Fluctuating Nature of Light-Enhanced d-Wave Superconductivity: A Time-Dependent Variational Non-Gaussian Exact Diagonalization Study, Physical Review X 11, 041028 (2021)
- Wang et al. Light-Enhanced Spin Fluctuations and d-Wave Superconductivity at a Phase Boundary, Physical Review Letters 120, 246402 (2018)